Dissolved oxygen, or DO, refers to the amount of free, non-compound oxygen in water or any other liquid. The measurement of dissolved oxygen is crucial when assessing water quality, pollution control, and treatment processes. This article reviews current methods of measuring dissolved oxygen, why these measurements are necessary, and the diverse applications that stem from this precise analysis.
Methods of Measuring Dissolved Oxygen
Measurement of dissolved oxygen is typically monitored in percentage air saturation (% sat) or milligrams per liter (mg/L). Moreover, there are several measurement methods, with some proving more advantageous in certain situations.
Winkler Method
The Winkler method, developed by a Hungarian chemist, L.W. Winkler, in 1888, is one of the earliest methods but remains relevant today. It is a titrimetric method involving a series of chemical reactions in a water sample to determine the DO level. Typically, the measurement process requires a specialized BOD bottle, which has a design that allows it to seal without trapping air inside. The water sample for testing is collected into the BOD, and reagents such as manganese peroxide solution and sodium hydroxide solution are added in proportion to the test sample. This reaction produces a precipitate of manganese hydroxide (II). If the sample does not contain water, the precipitate is white, as the reaction below shows.
Mn2++2OH-MnOH2 White precipitate
However, if DO is present in the test sample, it reacts to form a brown precipitate.
MnOH2+1/2O2MnOOH2 Brown precipitate
Next, the brown precipitate is dissolved in an acid in the presence of iodide ions to release iodine (I2) according to the amount of DO. Finally, the iodine released is titrated with sodium thiosulfate to determine the exact amount of oxygen.
MnOH2+2I-+4H+Mn2++I2+3H2O
I2+2S2O32-2I-+S4O62-
Although the Winkler method remains a recognized standard for DO analysis, it introduces the possibilities of human error, sample contamination, inaccuracies, and interferences. Several modifications have been developed to improve accuracy, but titrations remain cumbersome to execute, especially in the field. This is why new technologies focus on quicker and easier calculation methods while maintaining accuracy.
Electrochemical Method
Whether testing in the lab or in the field, the use of electrochemical sensors is a popular way of taking dissolved oxygen measurements. These sensors operate on the principle of oxygen consumption at an electrode surface, be it galvanic or polarographic. Both galvanic and polarographic DO sensors utilize two polarized electrodes – an anode and a cathode – in an electrolyte solution.
A thin, semi-permeable membrane or diaphragm separates the electrodes and electrolyte from the test sample. When taking measurements, the DO diffuses across the diaphragm at a rate proportional to the partial pressure of oxygen in the sample. The DO is then reduced and consumed at the cathode, thus producing a directly proportional electric current to the oxygen concentration. This current flows from the cathode to the anode via the ions in the electrolyte and relates to the partial pressure of oxygen as follows:
id=4×FPmtApO2d
In the equation, the terms id, F, Pm(t), A, pO2, and d represent the current produced, Faraday’s constant, permeability of diaphragm as a function of temperature, surface area of cathode, partial pressure of oxygen, and diaphragm thickness respectively.
Stirring these sensors in the sample is necessary until the DO readings no longer increase. This is because the measurement process depends on flow due to the consumption of oxygen molecules. As such, a no-flow situation can produce an artificially low dissolved oxygen measurement.
Galvanic Sensors
Galvanic sensors will self-polarize after being placed in an electrolyte solution. They do not require warm-up time and can operate without externally applied potential. Common material for the anode is zinc or lead, while the cathode is silver or another noble metal. Because the cathode is inert, it does not interfere with the reaction. Instead, it serves only to pass on electrons. Thus, the anode is oxidized, and oxygen is reduced at the surface of the cathode, with the overall reaction as follows:
O2+2H2O+2Zn→2ZnOH2
The zinc hydroxide produced by this reaction is precipitated as a white solid at the tip of the sensors but does not coat or consume the electrolyte. Over time, build-up can become excessive and interfere with the ability of the ions to carry current between the anode and cathode. At this point, it is necessary to replace the electrolyte solution.
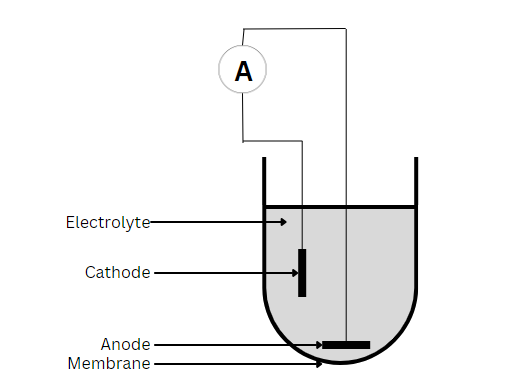
Polarographic Sensors
Polarographic sensors have a setup similar to that of the galvanic sensor but use a silver anode alongside a noble metal like gold or platinum as the cathode. As a result, it needs the application of an external potential (0.4 to 1.2V) to reduce oxygen from the cathode to the anode. Also, most applications require a warm-up period of 5 to 60 minutes. As the silver anode is oxidized, a dark AgCl coating becomes noticeable. However, this occurs only when DO measurements are taken, so the sensor will degrade slowly. This degradation process is a lot slower than that of a galvanic sensor, where the anode precipitates constantly. Over time, as this degradation occurs, the readings will be unstable and unusually low, thus necessitating maintenance to restore sensor performance.
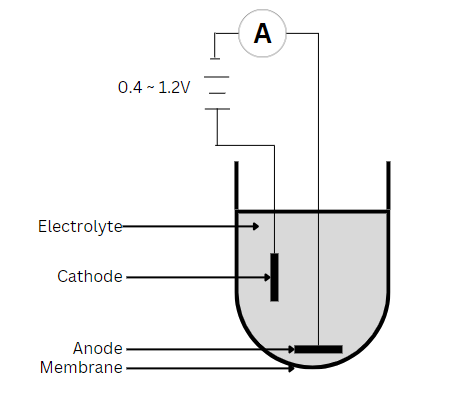
Optical Method
Rather than measuring reactions through the electrochemical sensors, optical sensors measure the interactions between dissolved oxygen and certain luminescent dyes. The measurement process begins with exposing the dyes to an incident light. As a result, the molecules of the dyes become excited and will emit light with longer wavelengths as their electrons return to the average energy state. If DO is present, the wavelength of the emitted light will be limited or altered due to the interactions between oxygen and the dyes. The frequency, intensity, and decay of the emitted light are related to the partial pressure of oxygen and can be used to determine its magnitude.
Optical DO sensors require minimum maintenance and are ideal for continuous measurement or long-term applications. They are generally more accurate than electrochemical sensors, mainly because they will not be affected by hydrogen sulfide or other gasses that can impact the performance of electrochemical sensors. In addition, they are also capable of measuring DO at concentrations much lower than the detection limits of the electrochemical sensors. The significant disadvantages of the optical DO sensors include higher power consumption, slower response time, and accuracy dependent on humidity.
Colorimetric Method
This method involves using colorimetric reagents that can react with dissolved oxygen to produce color changes proportional to DO amounts. A spectrophotometer, colorimeter, or a simple comparator commonly determine the color changes. Two common variations of the colorimetric method include Indigo Carmine and Rhodazine D.
The Indigo Carmine forms a blue-colored solution with DO and can measure its concentrations between 0.2 and 15 ppm. This measurement will be interfered with by the presence of ions such as ferrous, nitrite, and hydrosulfite. Rhodazine D will produce a rose-colored solution with oxygen and can detect much lower DO concentrations at the ppb level. The presence of oxidizing agents such as chlorine, ferric, and cupric will affect its measurement results.
Why Measure for Dissolved Oxygen?
Accurate dissolved oxygen measurements offer a window into the dynamic health of aquatic environments. If the DO levels are low, it could indicate pollution, eutrophication, or excessive microbial activities, which will jeopardize the well-being of aquatic organisms. Conversely, having high oxygen levels could signify the presence of algae or the effects of aeration devices.
Applications
Dissolved oxygen measurement is widely applied in several fields, including:
- Environmental Monitoring: DO measurements are fundamental in monitoring the impact of human activities on water bodies. For example, wastewater treatment plants and regulatory bodies rely on these measurements to assess compliance with environmental standards and identify sources of pollution. Testing DO in these plants helps in understanding the biodegradable organic matter and the biological oxygen demand. Both parameters are indications of the general water quality.
- Aquaculture Management: Maintaining optimal dissolved oxygen level is important for the health and growth of aquatic organisms. Continuous monitoring allows aquaculturists to adjust aeration and feeding rates, preventing oxygen-related stress and mortality.
- Oceanography and Limnology: Researchers in oceanography and limnology utilize DO measurements to evaluate nutrient cycling, oxygen dynamics, and the effects of climate change on aquatic ecosystems. These insights contribute to a broader understanding of global environmental dynamics.